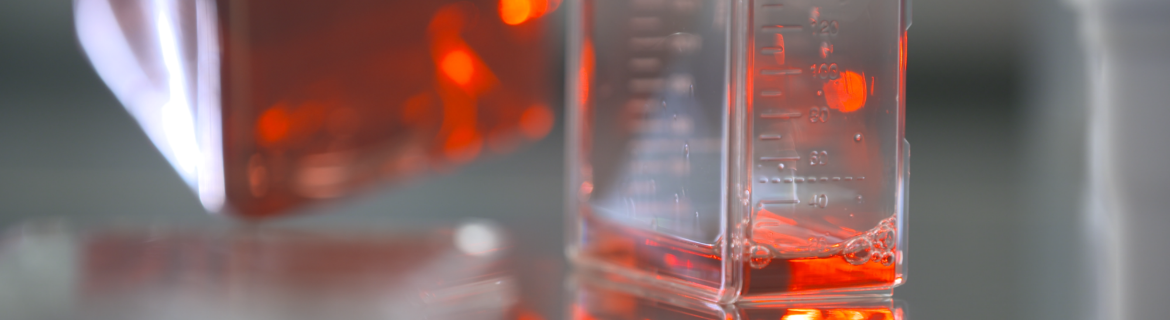
Emmanuelle Charpentier and Jennifer Doudna were awarded the 2020 Nobel Prize in Chemistry for developing the method. Researchers at the University of Stuttgart are using the technology in a number of different ways.
CRISPR-Cas9 gene scissors can be used to cut the DNA of plants, animals and microorganisms with pinpoint accuracy, after which individual DNA building blocks can be exchanged, or entire gene segments can be inserted at the intersection as required. Hopes are hight that this will pave the way to such things as the development of fungus-resistant corn, pigs with greater muscle mass or new cancer therapies. Eventually, it may also be possible to cure hereditary diseases.
Ever since the 1970s, methods of altering genetic material have existed but all of which have been too imprecise, time-consuming and expensive until recently. For example scientists exploited the fact that the cellular apparatus sometimes incorporates inserted genes rather than an existing gene variant. It was usually necessary to carry out crossbreeding experiments for many years until animals were produced with the new genes in all of their body cells.
Beginnings of the gene scissors
Gene therapy trials have often involved the use of attenuated viruses able to insert any piece of DNA into the genome, but in an uncontrolled manner and at random locations, which could result in the unintentional interruption of important genes, such as those that protect against cancer.
The first gene scissors, such as zinc-finger nucleases and later TALENs sent the research community into a state of euphoria at the turn of the millennium, because these were the first ever precise surgical gene tools. However they were never widely used: “These DNA-cutting enzymes have to be redesigned for each gene locus at which they are targetted, which is no trivial matter,” Prof. Albert Jeltsch explains. Jeltsch, who heads up the the Department of Biochemistry at the University of Stuttgart’s Institute of Biochemistry and Technical Biochemistry (IBTB) has been experimenting with gene scissors since their infancy.
The research field is exploding
The CRISPR-Cas9 method, by contrast, requires no complex protein design: all it needs is a short snippet of RNA containing the transcript of the target section of DNA to direct the DNA-cutting enzyme Cas9 to where it is required. “The sequence of this RNA probe then spits out a piece of online software for me,” Dr. Cathrin Hagenlocher of the Institute of Cell Biology and Immunology (IZI) explains, “which I can then purchase for less than 10 euros. This is a matter of five minutes if I know exactly where Cas9 should cut.”
Hagenlocher was working on her doctoral thesis when Charpentier and Doudna published the method in 2012. “I grasped the theory back then and thought, I want to use this technique too,” the cell biologist explains. Four years later, she immersed herself in the reams of protocols already available on the Internet at the time and established the new technique within her own working group.
We wanted to find out why some cancer cells are resistant in order that we might eventually be able to trigger the cell death of tumor cells as part of our cancer therapy.
Dr. Cathrin Hagenlocher
One of her students was looking into a signaling pathway in colon cancer cells that can force them into programmed cell death, but does not always respond to incoming self-destruct signals on the surface of the cell. “We wanted to find out why some cancer cells are resistant in order that we might eventually be able to trigger the cell death of tumor cells in a targeted manner as part of our cancer therapy,” says Hagenlocher, who goes on to say that the Institute is now able to produce gene knockout cell lines in which CRISPR gene scissors are used to destroy certain genes.
CRISPR-Cas is how bacteria defend themselves against viruses, CRISPR being the name of certain sections of the bacterial genome, in which the bacterium incorporates and stores specific sections of viral DNA from viruses that have infected it. In the event of a reinfection by the same virus, the bacterial enzyme Cas uses the selected section to recognize the genetic material of the hostile virus and then cuts the viral material at the exact site it has “memorized”, thus rendering the virus harmless. The bacterial system can easily be hijacked in the laboratory to perform targeted genetic modifications in all kinds of organisms.
“It is more complicated to insert something into the genome.” This is done by, for example, introducing the desired piece of DNA into the cell and allowing the cell's own repair system to insert it at the fracture point. Some members of the Institute have already successfully inserted known cancer mutations into cellular genetic material so that they can analyse them with greater precision. “The field is just exploding and there is an amazing number of modified forms of the original CRISPR-Cas9 technique,” says Hagenlocher.
Surprising findings thanks to CRISPR
The method can also be used for such things as delivering markers or proteins to specific gene locations, by deactivating the Cas9 enzyme to prevent it from cutting the DNA. Another research group led by Prof. Jörn Lausen, head of the Institute of Industrial Genetics, uses specific RNA probes to target Cas9 enzymes, for example, genes which usually control cell division and differentiation but which become misregulated in cancer. “Our goal is to identify the proteins that bind to the gene in which we are interested,” Lausen explains. These could be transcription factors needed to read genes. Yet so-called epigenetic enzymes also bind to them, which add or remove methyl groups from DNA, thereby switching certain genes on or off.
The scientists first slice up the complete genetic material of cancer cells to detect these proteins. They then fish out only those bits of DNA marked with the Cas9 enzyme from this DNA soup together with the proteins that bind to them. “If, for example, we find an epigenetic enzyme bound to a tumor suppressor gene, we could use inhibitors to reactivate the gene and inhibit the enzyme thereby forcing the cancer cell to self destruct. Inhibitors of this kind are currently being developed for clinical application around the world,” Lausen explains.
Basic researchers had only been able to confirm their hypothesis that protein X binds to gene Y using previous methods. “But with the CRISPR method,” the professor of genetics explains enthusiastically, “I can just see what I can find, which is usually something I never would have expected.”
With the CRISPR method, I can just see whatever I find.
Prof. Jörn Lausen
Albert Jeltsch is also working on epigenetic gene regulation at the IBTB. For the first time, the biochemist now hopes to directly couple a deactivated Cas9 enzyme with an epigenetic enzyme and test it in rats in a project for which he and another Tübingen-based researcher have applied. Their objective is to target and methylate, thereby switching off, a gene that is mutated or overactive in certain patients suffering from Parkinson's disease. “The benefit,” as Jeltsch explains, “is that only the epigenetic markers but not the genetic material is changed.” The gene would be irretrievably destroyed using the basic CRISPR-Cas9 method and the DNA damage could even cause cell death in some cases.
The Lego-Principle: Combine whichever building blocks you like
Fundamentally, inactive gene scissors can be used to combine any amount of building blocks, just like a set of Lego and assemble them to form completely new tools. Jeltsch and his colleague Dr. Pavel Bashtrykov, for example, have developed and patented a methylation sensor that enables the first live microscopic observations of how genes are switched on or off in individual cells during the cell division process.
To achieve this, a component of a fluorescent dye is coupled with inactive gene scissors – currently the CRISPR- Cas gene scissors – and transported to the target gene site. The other half of the dye is coupled with a protein domain that is able to recognize methylation sites. Whenever the two dye components are in close proximity, they glow thus proving that a specific gene has been switched off.
Given the many possibilities, a debate on medical ethics has been raging in scientific circles for a while now. In particular, any intervention in the germline is considered to be controversial whereas gene therapies for adults for severe or previously incurable diseases are viewed in a less critical light because, as Jeltsch explains, “an unborn child cannot comment on the question whereas an adult can.”
The benefit is that only the epigenetic markers but not the genetic material is changed.
Prof. Albert Jeltsch
However, CRISPR technology is also raising concerns among the public: “These concerns,” as Jörn Lausen reports following a panel discussion, “extend to suggesting that it might be possible to breed super soldiers who could take over the world.” As co-inventor Jennifer Doudna wrote in Nature in February 2020, there are still many unanswered questions about the long-term efficiency and safety of the CRISPR process in humans. Even though the precision tool sometimes makes a cut at the wrong position, the technology is constantly being improved.
This technology has already been used in the lab to produce numerous useful plants and animals with new properties None of these organisms is currently on the market. They are also considered to be “genetically modified” within the EU and have to go through an elaborate approval process. Clinical trials are currently being conducted in humans to test CRISPR therapies for cancer, HIV, inherited blood disorders as well as for a rare congenital blindness condition. In the majority of countries, genetic modifications in germline cells that are passed on to offspring are prohibited, but a set of twins, who were genetically engineered using CRISPR-Cas were born in China in 2018, for which scientist He Jiankui and two of his colleagues received jail sentences and heavy fines.
Researchers bear responsibility for genetic changes
“For us as basic researchers,” says Prof. Dr. Markus Morrison, director of the Stuttgart Research Center Systems Biology and head of University of Stuttgart’s Institute of Cell Biology and Immunology, “this type of ethical question does not arise, as we don’t cure patients or intervene in the germline of human embryos.” Nevertheless, he continues, anyone who genetically modifies cell lines and animals used as model systems in research carries a certain responsibility. “Every genetically modified cell line or animal experiment requires a risk assessment and a decision by an independent ethics committee,” Morrison stresses.
CRISPR-Cas is accelerating research at a tremendous rate and is set to become the next standard in molecular biology.
Prof. Markus Morrison
Whatever the outcome of the debate about the use of gene scissors on humans, Morrison is convinced that: “CRISPR-Cas is accelerating research at a tremendous rate and is set to become the next standard in molecular biology.”
Text: Helmine Braitmaier
Prof. Markus Morrison, Institute of Cell Biology and Immunology
Mail, Fon: +49 711 685 66987